Water-Energy-Food (WEF) Nexus
Reaching the UN’s Sustainable Development Goals (SDGs), including those on Zero-hunger (SDG 2), Clean water and sanitation (SDG 6) and Affordable and clean energy (SDG 7), require substantial, if not transformative, efforts across the actor landscape.
As both food and energy (e.g. hydro-electric, nuclear and thermal plants) require water for production, it has been recognised that when the use of resources is not well planned and managed there can be conflicting trade-offs between water-energy-food (WEF). For example, allocating more water to produce more food implies that less will be available for energy or other uses and vice versa (Figure 1).
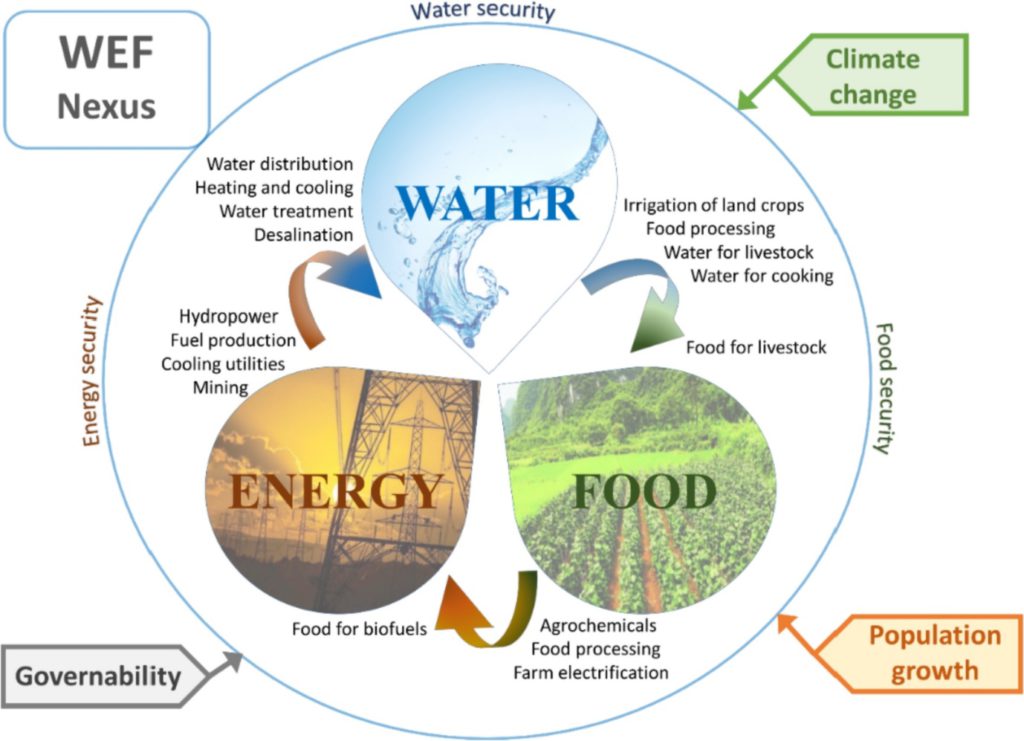
Figure 1. Summary of the water-energy-food (WEF) Nexus. Source: Mahlknecht et al, 2020
These trade-offs constitute the so-called WEF trilemma, whereby if water management is not addressed in a more holistic way, the water availability for ecosystems, agriculture, people and services will decrease and conflicts may arise. More specifically, it is important to consider the needs of all elements of the socio-ecological system, with a special emphasis on maintaining healthy ecosystems and a functioning water cycle with aquifers getting recharged at a rate equal or faster than the water usage. And since the energy and agriculture sectors are the main contributors to greenhouse gas emissions, which in turn drive climate change also affecting rainfall patterns, such trade-offs become increasingly important. Additionally, water stress is also increasing globally (Figure 2) due to overexploitation and contamination of aquifers and deforestation, which if not addressed properly it can worsen because of long-term global trends including population growth, income growth (possibly leading to more use of resources), and climate change.
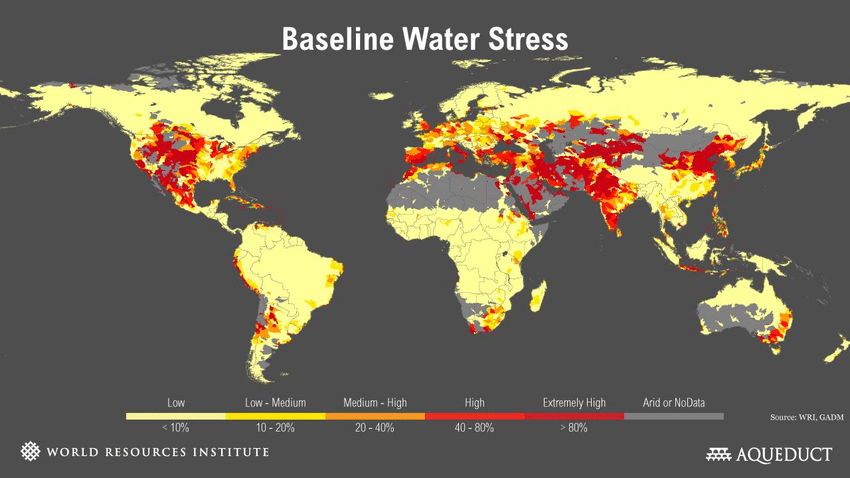
Figure 2. Current global water stress, showing areas with high and extremely high stress outside of historical arid regions. Source: WRI –Water Risk Atlas
To understand and find solutions to this trilemma, it is necessary to adopt a more holistic approach, as stated above. Two established approaches to tackle this issue are: Nexus Perspective and Systems Resilience. While the essence of the nexus approach is about interconnections between water, energy and food systems, resilience is about the capacity of a system to respond to threats and retain its ability to deliver benefits (Stringer et al 2018).
Nexus thinking highlights relationships, interdependencies, and the need for integrated decision-making across sectors. It is most commonly used to examine WEF interactions within risk-based decision processes. To explain the nexus in its simplest form, water is needed to generate energy, energy is needed to supply water, energy is needed to produce food, food can be used to produce energy, water is needed to grow food, while food transports (virtual) water, often using energy (Stringer et al., 2018 and references therein, Figure 1).
Whereas resilience has been defined as the ability of a social-ecological system to cope with shocks and stresses by responding or reorganizing in ways that maintain its essential functions, identities and structures, while also maintaining the capacity for adaptation, learning, and transformation (Stringer et al. 2018 and references therein). The Nexus thinking finds its origins in environmental policy studies and public debate on natural resources management, while resilience has precursors in science debates on sustainability and systems thinking.
Both approaches can provide key insights and on their own have some limitations. However, it is important to consider synergies and trade-offs between subsystems within the larger WEF nexus and at the same time consider cross-system resilience linkages.
One of the key lessons from resilience thinking is that we need to understand the implications of cross-scale dynamics or interventions that operate at different scales of the system, where the system is understood as an interconnected whole. In contrast, when each issue is analysed independently it is highly likely that the multiplicity of feedbacks and interdependencies that jointly affect their sustainability will be overlooked (Hogeboom et al 2021).
There is also an integrated nexus-resilience thinking framework that could be a good base to further understand this topic and to help find feasible solutions (Stringer et al. 2018). It is based on three principles:
- Unpack relationships and interactions in Socio-Ecological Systems
- Traverse connections between temporal and spatial scales, sectors, stakeholders, etc
- Share knowledge, learning, and experience to empower stakeholders involved.
Common threats for WEF systems are:
- Climate change: altering rainfall patterns, storms, cold waves, and droughts
- Habitat loss and soil erosion and soil impoverishment (that also affects water retention and release of Green House Gases from bare soils)
- Resource supply limitations including water, nutrients and land (Figure 3)
- Human migration
- Urban transformation
Energy generation and WEF nexus
Energy generation is already experiencing competition in the use of resources with other sectors. This is especially true for the water sector, as water is used for energy generation as with hydropower and thermal power cooling, but it is also critical for ecosystems’ health, agriculture (e.g. irrigation), domestic use (e.g. drinking water supply), recreation (e.g. tourism, fishing), and flood control.
Some energy technologies that reduce greenhouse gas emissions, such as carbon capture and storage (CCS), concentrated solar power, and geothermal generation, have the potential to increase energy’s water intensity; others, such as photovoltaic (PV) solar power and, especially, wind power require considerably less water. Though there can be a strong link between energy and water efficiency in energy technologies, most evaluation criteria do not incorporate water use or water performance metrics (USA Energy Review, 2020).
Climate change is already translating into measurable impacts on the water cycle, and it is anticipated that these will be even more prominent in the future. Water availability may decrease in places, due to more intense and longer droughts, while in other areas flash flooding could become more frequent. It is therefore apparent how information on water resource availability, weeks to months to decades ahead, needs to be an integral component of energy operations and planning. Designing technologies and optimizing operations for improved water performance can have both energy and water benefits (USA Energy Review, 2020).
Weather, Hydrological and Climate Services for water prediction from the nexus perspective
Reservoirs must be operated based on the seasonal cycle of water inflows which, historically, have been forecasted based on climatological data, often using analogue methods. However, with current changes in precipitation and increasing droughts, historical data to forecast future water volumes is less and less accurate. Significant progress in monthly to seasonal forecasts has been made in the past decade, but forecasting skill remains moderate to low in some regions and periods of the year, and forecasting products are still not tailored enough to be used operationally, even if some recent projects, like the EU SECLI-FIRM project, have made a clear demonstration of the potential of seasonal forecasts for hydropower applications (WMO SG-Ene, 2022). A dynamic approach, constantly adjusting water allocation for each sector to improve the utilization efficiency of water resources has been suggested to overcome long-term uncertainty (Zhang et al., 2018)
The main difficulty in modelling hydric resources is the associated uncertainty of rainfall forecasting. Prediction systems use satellite measurements, meteorological and hydrological forecasting models, water supply-demand prediction models, and decision support tools. Advances in sensors and instrumentation as well as computational capability, open new possibilities for the field of hydrometeorology, although there is still a lack of adequate knowledge to accurately forecast extreme hydrometeorological events.
In the agricultural community, hydrometeorological modelling is used to predict the timing and amount of precipitation to forecast crop yields and warn of freshwater shortages that might affect irrigation and production. Today’s satellite data provides global precipitation estimates over land that can be incorporated into forecast models.
Another application where satellite observations are playing a significant role is connected to water resource managers, who rely on accurate precipitation measurements to monitor freshwater resources necessary for human activities. This includes public consumption, irrigation, sanitation, mining, livestock and powering industries. Global observations of precipitation from satellites allow for a better understanding and prediction of changes in freshwater supply (WMO SG-Ene, 2022).
References
- Hogeboom, Rick J. and Borsje, Bas W. and Deribe, Mekdelawit M. and van der Meer, Freek D. and Mehvar, Seyedabdolhossein and Meyer, Markus A. and Özerol, Gül and Hoekstra, Arjen Y. and Nelson, Andy D. 2021 Resilience Meets the Water–Energy–Food Nexus: Mapping the Research Landscape, Frontiers in Environmental Science Vol 9 https://www.frontiersin.org/article/10.3389/fenvs.2021.630395, doiñ10.3389/fenvs.2021.630395
- Jürgen Mahlknecht, J., González-Bravo, R., Loge, F. 2020. Water-energy-food security: A Nexus perspective of the current situation in Latin America and the Caribbean. Energy, Vol. 194, 116824, doi.org/10.1016/j.energy.2019.116824.
- USA Energy Review, 2020 Transforming the Nation’s Electricity System: The Second Installment of the Quadrennial Energy Review. Summary for Policymakers. USA https://www.hsdl.org/?abstract&did=797992
- Stringer, L. C., Quinn, C. H., Le, H. T. V., Msuya, F., Pezzuti, D. R., Dallimer, M., et al. (2018). A New framework to enable equitable outcomes: resilience and Nexus approaches combined. Earths Future 6, 902–918. doi:10.1029/2017ef000694
- WMO SG-Ene. 2022. Weather and Climate Services in the context of the global Energy transformation, In: Guidelines of the WMO Commission for Weather, Climate, Water and Related Environmental Services and Applications- Study Group on Integrated Energy Services, Integrated weather & climate services in support to net zero energy transition.
- Zarco-González, Z., Monroy-Vilchis, O., Antonio-Némiga, X., & Endara-Agramont, A. R., 2022. Land use change around hydroelectric dams using Landsat multi-temporal data: a challenge for a sustainable environment in Mexico, Geocarto International, 37:21, 6375-6390, DOI: 10.1080/10106049.2021.1933212
- Zhang, X. H Li, H-Y, Deng, Z, Ringler, C., Gao, Y., Hejazi, M, Leung, R. 2018. Impacts of climate change, policy and Water-Energy-Food Nexus on hydropower development Renewable Energy, 116: 827-834